Brain Studies Reveal Mechanisms Of Voluntary Control Of Visual Attention
Neuroscientists at Duke University have mapped the timing and sequence of neural activations that unfold in the brain when people focus their attention on specific locations in their visual fields.
The findings may point the way for clinicians to address attention-related problems, said study team member Marty Woldorff, associate director of the Center for Cognitive Neuroscience and an associate professor in psychiatry.
"There are a number of clinical syndromes where attention is dysfunctional, including schizophrenia, autism and attention deficit-hyperactivity disorder," Woldorff said. "Moreover, attentional capabilities change during normal and abnormal aging."
The findings appear in the January 2007 issue of the journal Public Library of Science (PLoS) Biology. The research was supported by the National Institute of Mental Health and the National Institute of Neurological Diseases and Stroke.
In this study, the researchers combined information from two different technologies for measuring brain activity in order to provide insight into the basic mechanisms by which humans orient and focus their visual spatial attention.
"At every moment of our lives, we are deluged with sensory stimuli coming from multiple directions," Woldorff and study co-investigator Tineke Grent-'t-Jong wrote in the journal report. Grent-'t-Jong is a research associate at Duke's Center for Cognitive Neuroscience and a graduate student in psychopharmacology at the University of Utrecht in the Netherlands.
This sensory deluge encompasses "much more information than we can fully process," they said. "The critical function of attention allows us at each moment to continuously select and extract the most important information from this flood of sensory inputs."
An example of the kind of focused visual attention the researchers investigated is what happens as a motorist watches traffic in front of his car while also monitoring out of the corner of his eye the movement of a bicyclist pedaling on the side of the road. This example is illustrated on Woldorff's laboratory website at http://www.mind.duke.edu/faculty/woldorff/.
"When we direct our attention to such specific locations or events - in this case, the bicyclist off to the side - we tend to more quickly discriminate or detect changes there than at other locations in our visual field that are not so attended," Woldorff said.
Previous studies, including several by the Duke researchers, have associated such attentional processes with brain activity that occurs in what is called the "frontal-parietal attentional control network." This network comprises areas of nerve cells located in the front portion and the upper rear, or parietal, portion of the brain.
Previous studies also have indicated that areas of the visual cortex, located in the lower rear part of the brain, preferentially process information coming from locations or objects in the visual field where attention is directed.
Those studies have additionally suggested that the frontal-parietal network may induce this improved processing in the visual areas by means of some "biasing" or enhancing signal.
But "little is known about the timing and sequence of activations within this frontal-parietal network and their temporal relationship to any such biasing," the Duke scientists said in their report.
They said the uncertainty about such characteristics is due mainly to specific technical limitations of two of the main technologies currently used to monitor brain activity in humans: functional magnetic resonance imaging (fMRI) and event-related potential (ERP) recordings.
Although fMRI can pinpoint the locations of such brain activity by measuring changes in regional blood flow associated with increased neural activity, the technology is "very limited as to the timing information it can provide," Woldorff said. "The reason is that it takes multiple seconds for blood flow to change and to yield a measurable fMRI signal."
ERP recordings, which are extracted from electroencephalography (EEG) recordings made at the scalp of electrical activity in the brain, can reflect the brain's responses to sensory and cognitive events with millisecond precision, he said. But the electrical brain activations detected with ERPs are harder to trace back to their exact origins inside the brain.
To overcome these limitations, Grent-'t-Jong and Woldorff combined brain responses from the two technologies recorded in identical experiments.
"To effectively understand how the brain accomplishes cognitive functions, it is critical to be able to delineate both the brain regions that are involved and the timing and sequence of their activations," Woldorff explained.
In the experiments, participants were asked to keep their gaze directed toward the center of a computer screen. They then were presented with a series of four-second trials, each beginning with the appearance of an "instructional" letter cue that the participants had been coached on how to interpret. For example, participants might be shown an "L" cue instructing them to focus their attention "covertly" - that is, without moving their eyes - to a location on the left side of the screen.
Most such letter cues instructed participants to direct their attention to a specific location on the screen and be alert for a possible faint dot "target" that might appear there during the following second or two. During some trials, however, the cue directed participants not to orient their attention to a particular location because no target would be appearing on that trial.
In all of the trials, participants had to decode and interpret the meaning of the instructional letter cue. But only in response to attention-directing cues did participants also orient their visual attention. As a result, the researchers were able to separate brain activity specifically related to attentional orienting from activity associated with general cue processing.
The fMRI findings - first reported in January 2004 in the Journal of Cognitive Neuroscience - showed that lateral parts of the frontal-parietal network were more involved in "cue interpretation," Woldorff said. Meanwhile, regions of the network closer to the brain's midline were more specifically involved in "attentional orienting."
But since fMRI recordings cannot delineate the relative timing of these activations, the researchers launched their current study, which followed the same experimental design used in the earlier study but incorporated time-sensitive ERP recordings.
The study revealed that both the attention-directing cues and the "no-target-will-appear" cues evoked similar electrical activity during the first 300-400 milliseconds following their appearance on the screen. After that, electrical brain activity for the "no-target-will-appear" cues quickly ebbed away, whereas activity for attention-directing cues continued for an extended period.
According to Grent-'t-Jong and Woldorff, these results indicated that the initial electrical response up to 400 milliseconds mainly reflected general cue processing activity. In contrast, the later continuing electrical activity was due primarily to the participants' following the attention-directing cue's instruction to covertly orient attention to the left or right side of the screen.
The prolonged response to the attention-directing cues began initially over only the front part of the scalp, later extending to parietal scalp areas over the top and upper rear of the head. This pattern of ERP activity suggested an initial start of attentional orienting in frontal areas only, followed by joint activity in both frontal and parietal attentional control brain areas, Woldorff said. Additional computer analysis combining both the fMRI and ERP recordings confirmed this sequence.
The brain activity recordings also indicated that the attentional orienting activity in the frontal-parietal network regions was followed by increased activity in the visual cortex, prior to the occurrence of any visual target stimulus.
Woldorff said activity in the visual cortex before the target is even presented seems to reflect a preparatory biasing of those sensory brain regions to facilitate processing for a possible upcoming target. Moreover, this biasing activity was exclusive to the part of visual cortex corresponding to the region of space where the upcoming target would appear.
These results indicate that specific regions of the frontal cortex activate first, followed by the parietal cortex, in a sequence that initiates the shifting of the focus of visual attention in space, according to Woldorff. "Those frontal and parietal areas then seem to work together to exercise control over the sensory inputs at the early stages of the visual pathways," he said.
This control appears to prime the appropriate brain areas to more fully process certain aspects of the visual scene, he said. In this particular study, the priming was for a certain region of the visual field. In other circumstances, the brain might be primed for a certain color or shape.
In the case of the researchers' hypothetical motorist and bicyclist, "such enhanced processing might then enable the motorist to make a faster accident-avoiding response if the bicyclist suddenly made an unexpected movement toward the car," Woldorff said.
"Or, once upon a time, it may have helped our prehistoric ancestors forage for berries while carefully maintaining attention on a distant, hopefully not too hungry, lion."
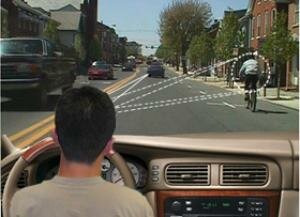
"Action at the corner of our eye can become a focus of our attention, as illustrated by the bicyclist in this Center for Cognitive Neuroscience graphic. (Courtesy of Kenneth Roberts)"
Source: Duke University
|